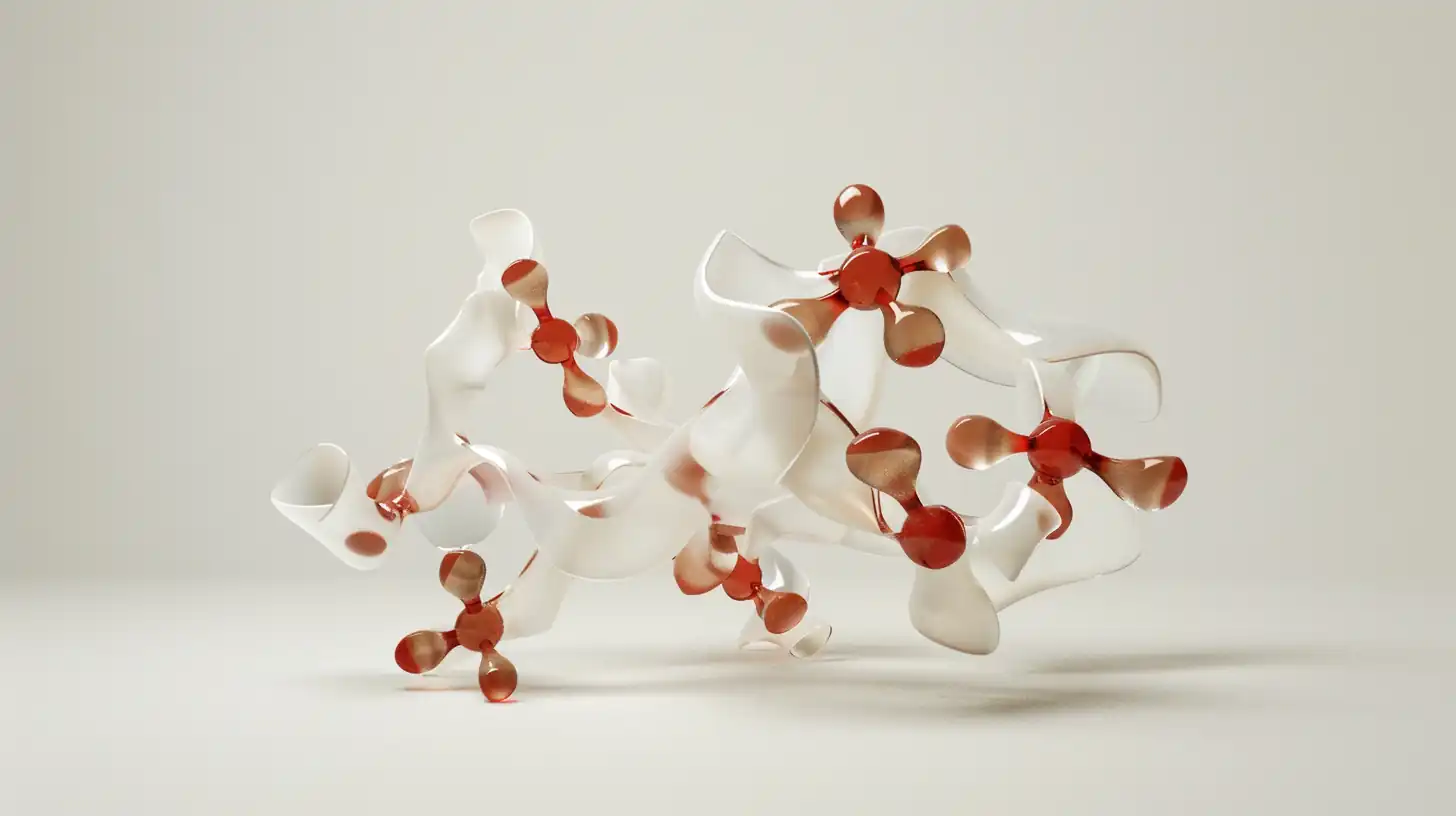
A ribosome works like a factory, but without the right delivery, production stops. In cells, that delivery is handled by a small RNA molecule called tRNA. Without it, protein synthesis can’t move forward, no matter how complete the instructions are.
In this guide, you’ll learn what tRNA is, how it connects codons on messenger RNA with the correct amino acids, and how the anticodon region ensures accuracy. We’ll break down its structure, pairing with codons, the loading of amino acids, and its function during translation.
tRNA: Quick Summary
Do you just need the basics? Here’s a simple explanation of what tRNA does:
🟠 tRNA is a short RNA molecule that carries a specific amino acid to the ribosome during protein synthesis.
🟠 Each anticodon on the tRNA pairs with a matching codon on the mRNA to place the correct amino acid in the chain.
🟠 Enzymes called aminoacyl-tRNA synthetases attach the right amino acid to each tRNA through a two-step chemical reaction.
🟠 Modified nucleotides like inosine and pseudouridine help stabilize transport RNA structure and improve codon recognition.
🟠 During translation, tRNA moves through the A, P, and E sites of the ribosome to form peptide bonds and grow the protein.
What is tRNA?
You’ll find tRNA in the cytoplasm, where it connects genetic code to protein building. It works during translation—when ribosomes read messenger RNA (mRNA) and form proteins. Each tRNA brings one specific amino acid and matches it to a codon on the mRNA strand.
The structure of tRNA looks like a cloverleaf. One end has an anticodon—a set of three RNA bases that pairs with a codon on mRNA. The other end holds the amino acid that fits that codon. Loops and folds help the molecule stay stable and fit into the ribosome.
Part of tRNA | Function |
Anticodon | Matches the mRNA codon with correct base pairing |
Amino acid site | Carries the amino acid to the ribosome |
D loop | Helps with folding and enzyme recognition |
TψC loop | Helps attach the tRNA to the ribosome |
Acceptor stem | Connects the anticodon region to the amino acid site |
How tRNA Binds Codons During Translation
Codon-anticodon pairing
A codon is a sequence of three nucleotides on the mRNA. A transport RNA (tRNA) carries a matching anticodon that pairs with it. The base-pairing follows simple rules: A pairs with U, and C pairs with G. The codon and anticodon bind through hydrogen bonds, which makes the decoding accurate. Each anticodon ensures that the correct amino acid is placed in the growing protein chain.
Examples of codon and matching anticodon pairs:
- Codon: AUG → Anticodon: UAC
- Codon: GCU → Anticodon: CGA
- Codon: UUU → Anticodon: AAA
- Codon: CCG → Anticodon: GGC
- Codon: AAG → Anticodon: UUC
Ribosome and tRNA movement
Translation happens in the ribosome, where each transport RNA binds in one of three sites: A-site, P-site, or E-site. The A-site accepts the incoming tRNA. The P-site holds the tRNA that carries the growing peptide chain. After the peptide bond forms, the ribosome shifts. The used tRNA moves to the E-site and exits. The next tRNA enters the A-site, and the process repeats until the protein is complete.
How tRNA Is Charged with Amino Acids
Enzymes that load amino acids
Aminoacyl-tRNA synthetases match amino acids with the correct tRNA. Each enzyme works with one specific amino acid and recognizes features of its tRNA, such as the anticodon or the acceptor stem. This pairing makes sure that the correct amino acid is used during translation. Most cells have 20 different synthetases—one for each amino acid.
Two-step process of charging
Step 1: Amino acid activation
The enzyme binds an amino acid and ATP. This creates a high-energy compound called aminoacyl-AMP and releases a phosphate group. The amino acid is now ready for attachment.
Step 2: Binding to tRNA
The enzyme transfers the activated amino acid to the 3′ end of the tRNA. This forms a bond between the amino acid and the sugar in the tRNA backbone. The charged tRNA now carries the amino acid to the ribosome, where it is used in protein synthesis.
Structure and Chemical Properties of tRNA
Folding patterns of tRNA
tRNA folds into a compact shape that helps it bind both the ribosome and mRNA. Its cloverleaf structure shows four regions: the acceptor stem, D-loop, anticodon loop, and TΨC loop. Each loop supports a specific function in translation.
In three-dimensional form, the cloverleaf folds into an L-shape. This arrangement places the anticodon and the amino acid attachment site on opposite ends. The shape helps transport RNA fit precisely into the ribosome.
Modified nucleotides
Once transcribed, tRNA undergoes chemical changes. These modifications improve structure and pairing accuracy. Nearly 10% of the nucleotides in each tRNA are altered after synthesis.
Common modified nucleotides:
- Inosine: pairs with multiple codons at the third position
- Pseudouridine (Ψ): stabilizes internal folding
- Dihydrouridine: improves flexibility in loop regions
- Methylguanosine: supports interaction with ribosomal RNA
- Thymidine: found in the TΨC loop, stabilizes the overall structure
These changes help tRNA function reliably in translation.
Transport RNA Variants in Eukaryotes and Prokaryotes
tRNA exists in both eukaryotes and prokaryotes, but there are differences in how it is processed and functions. In bacteria, tRNA genes are often organized in clusters. In eukaryotes, the genes are spread across the genome and require more complex processing.
In prokaryotes, a tRNA transcript may contain multiple tRNA sequences. Special enzymes then cut the long strand into individual units. These tRNA strands undergo basic modifications before they’re ready to function.
In eukaryotic cells, tRNA genes are transcribed in the nucleus by RNA polymerase III. The initial transcript, called pre-tRNA, goes through multiple processing steps. Introns—noncoding sequences—may be present and need to be removed. The ends of the molecule are trimmed, and a specific sequence, CCA, is added to the 3′ end if it’s not already encoded in the gene.
Modified nucleotides are added during or after these steps. Once complete, the mature tRNA is transported into the cytoplasm, where it takes part in translation.
These differences show how both systems solve the same task—producing functional tRNA—but follow slightly different steps to get there.
tRNA Genes and Redundancy in the Genetic Code
The genetic code has 64 codons, but most organisms have fewer than 64 different tRNA types. That’s because of codon redundancy. Several codons can specify the same amino acid. Cells use this redundancy to their advantage with a process called wobble pairing.
At the third position of the codon, base-pairing is more flexible. One anticodon can match with more than one codon. This allows fewer tRNA molecules to handle all possible codons. For example, a tRNA with the anticodon GAI (where I is inosine) can pair with codons CUU, CUC, and CUA, all coding for leucine.
This flexibility reduces the total number of tRNAs needed. Still, organisms have multiple tRNA genes for the same anticodon, especially for amino acids that are used often. These copies help maintain efficient protein synthesis.
In humans, some tRNA genes are also linked to mitochondrial function. Mitochondria have their own set of tRNA genes and make proteins using a slightly different genetic code. Errors in these mitochondrial tRNA genes can affect energy production in cells.
tRNA in Mitochondria
Besides the cytoplasm, tRNA is also found in mitochondria, where it supports protein synthesis specific to that organelle. Mitochondrial tRNA has a simpler structure compared to cytoplasmic tRNA. Some loops are shorter, and certain regions have fewer modified nucleotides.
Mitochondria have their own genome, which includes genes for 22 types of tRNA. These are enough to decode all 13 proteins that mitochondria make for themselves. However, these tRNA molecules don’t always follow the same pairing rules as those in the cytoplasm. For example, wobble pairing in mitochondria may occur at different positions or with less restriction.
Because of the streamlined mitochondrial system, a single tRNA may pair with more codons than usual. This simplification helps fit the coding requirements into a small genome, but it also means the system is more vulnerable to errors.
Mutations in mitochondrial tRNA genes have been linked to several inherited diseases. These conditions often affect tissues with high energy demands, such as nerves and muscles.
You can think of mitochondrial tRNA as a specialized version built to match the needs of its environment—efficient, compact, but sensitive to change.
Evolutionary Origin of tRNA
Scientists believe that tRNA evolved early in the history of life, possibly before ribosomes fully formed. Its ability to link nucleotide sequences with specific amino acids makes it a strong candidate for one of the earliest adapters in molecular evolution.
One theory suggests that tRNA originated from the fusion of two short RNA hairpins. These smaller RNA units folded into stable shapes and gradually combined into a structure like modern tRNA. Over time, this molecule took on the task of matching codons with amino acids.
tRNA’s structure has stayed consistent across species, from bacteria to humans. Its conserved shape and function suggest strong evolutionary pressure to keep it unchanged. Even in rapidly evolving organisms, the basic form of tRNA remains recognizable.
This evolutionary stability helps explain why translation works so reliably across all life forms. Once tRNA became efficient at its job, there was little reason to change it.
One-on-One Help with tRNA and Translation
Struggling to keep tRNA straight? You’re not the only one. The steps can feel like a maze—anticodons, codons, amino acid loading, A-site, P-site, E-site. A good chemistry tutor can break that down with you, slowly and clearly, so you’re not just memorizing—you’re actually seeing how it all fits together.
If you’re looking for help, search for “chemistry tutor Birmingham” or “tutoring chemistry Leeds” to find someone nearby. Plenty of students also go for online lessons, which can work just as well. A private teacher can guide you through tough parts like tRNA structure, pairing logic, or how enzymes load amino acids.
In one-on-one chemistry tutoring, you can ask every question you were too unsure to ask in class. No rushed lessons, no pressure to keep up. You go at your pace. Search for something like “organic chemistry tutoring online” or “private chemistry tutor for high school” and see who fits. You’ll come away more confident and actually ready to answer the test questions. Give it a try, book a session on meet’n’learn today.
Looking for more resources? Check out our Biology blogs for additional learning material. If you’re ready for extra help, a tutor can guide you through the most challenging topics with clarity and patience.
tRNA: Frequently Asked Questions
1. What is tRNA made of?
tRNA is made of about 70–90 ribonucleotides folded into a specific structure with modified bases.
2. Where does tRNA function in the cell?
tRNA functions in the cytoplasm during translation at the ribosome.
3. What is the anticodon on tRNA?
The anticodon is a three-nucleotide sequence that pairs with a complementary mRNA codon.
4. How does tRNA carry amino acids?
Each tRNA has an amino acid attached to its 3′ end by an aminoacyl-tRNA synthetase.
5. How many types of tRNA exist in humans?
Humans have around 48 different tRNA types based on unique anticodon sequences.
6. What connects an amino acid to tRNA?
An enzyme called aminoacyl-tRNA synthetase links the correct amino acid to each tRNA.
7. What is transport RNA’s structure?
Transport RNA has a cloverleaf secondary structure and an L-shaped 3D form.
8. Why does tRNA have modified nucleotides?
Modified nucleotides in tRNA improve folding, stability, and flexible codon pairing.
Sources:
1. NCBI
2. Britannica
3. Wikipedia
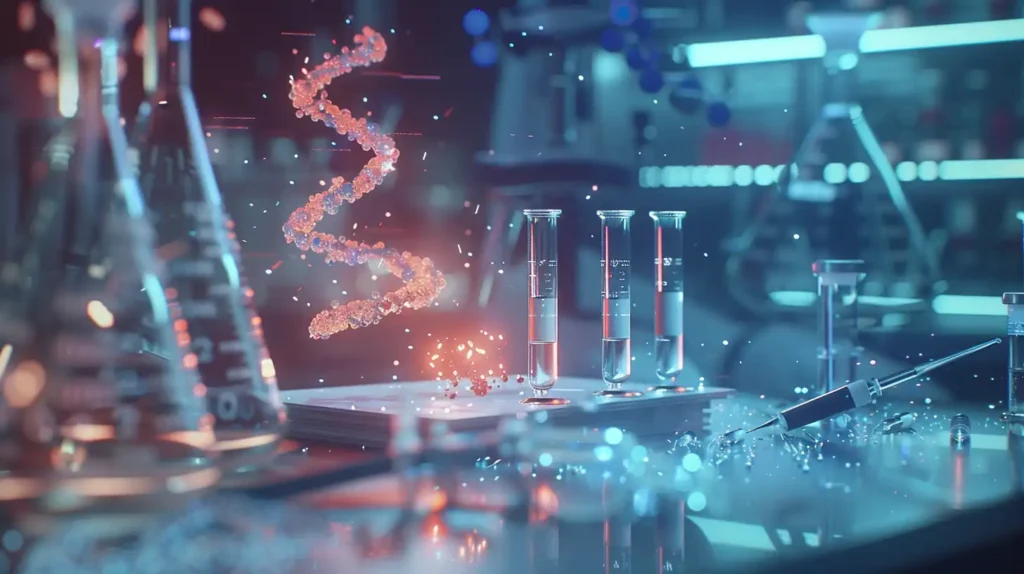